Contributed by Saad A. Akhtar
Fungi and Nematodes
Whenever people take the time out of their day to wonder about some of the awesome things living in the vast glory of nature, they generally think of every creature except fungi and nematodes. Observant readers will realize my post concerns fungi and nematodes.

Don’t look at me like that. Those were great opening lines.
But wait, don’t leave! I’m here to talk about interesting fungi and the nematodes that they eat! Yes, the fungi I’m talking about eat living, breathing nematodes, and they do so by laying out traps. I promise they’re really cool!
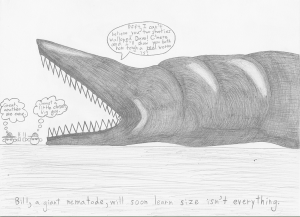
In fact, Bill learns how cool fungi are when he finds himself in a pit of lava moments after he mocks them.
(Note that the picture above can be enlarged by clicking on it.)
Fungi of the species Arthrobotrys oligospora normally get their grub by taking in nutrients from dead organic material just like other, typical species of fungi. However, when the fungi find that there is little nitrogen in their environment, they gain the ability to detect the concentration of ascaroside secreted from the nematodes into the soil. By the way, ascaroside is a molecule that regulates development and behavior in nematodes. In other words, the fungi can eavesdrop on chemical communication held among their nematode prey. Yes, I know what you’re thinking and you’re absolutely right…
“Indeed, the majority of our employees are carnivorous fungi. Why do you ask?” – an NSA spokesperson
Then, when the fungi find a nematode, they decide to eat those juicy, wriggling worms instead of sucking up nutrients from the soil.
“But how do immobile fungi catch something that can move?,” asks one hypothetical reader. Well, dear reader, the fungi create adhesive networks in response to the amount of nematodes they detect in the soil. You can think of these networks as spider webs in that they stick to prey and prevent them from moving.

Yeah, like we needed any other creature that acted like these things.
An Evolutionary Misconception
Now, you may be thinking that the ability to detect nematodes is something that fungi can obtain throughout their lifetime. However, this notion is incorrect as only a population of individuals can change over time, not an individual itself. In other words, evolution occurs over time in a population and is not instantaneous.
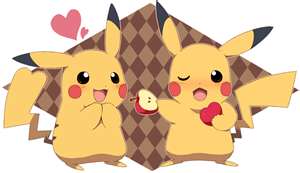
What I’m really trying to say is that living things aren’t Pokémon.
A conceivable example disproving this misconception would involve a population of fungi containing carnivorous and non-carnivorous fungi. The carnivorous fungi would be able to obtain food when nutrients in the soil are scarce, while the non-carnivorous fungi would not be able to do so and end up dying during those difficult times. Thus, there would be more carnivorous fungi that could pass on their genes and produce carnivorous offspring. Over time, the fungal population would consist mostly of carnivorous fungi rather than non-carnivorous fungi. As you can see from this example, the fungal population evolved and became carnivorous as a whole while individual non-carnivorous fungi did not suddenly gain the ability to become carnivorous. If you think about it a little bit, this conclusion really does make a lot of sense.
.jpg)
Um, sorry to shatter your worldview.
Coevolution
A rather important topic that this system touches on is coevolution. Coevolution is where two populations from different species influence the evolution of the other population. For instance, the A. oligospora fungi population is able to detect a certain form of ascaroside but if the nematode population evolves to secrete another kind of ascaroside molecule, the fungal population could also evolve in response to that change. Thus, the fungi and nematode populations coevolve. Note studied have not tested whether the nematode and fungi populations were actually evolving in response to one another, but researchers have considered it to be a real possibility.
See, I told you guys I had interesting stuff to say!

I even have a complimentary dog picture for you all on your way out! Isn’t life great or what?
For more information, see:
Hsueh, Y., Mahanti, P., Schroeder, F.C., & P.W. Sternberg. 2013. Nematode-trapping fungi eavesdrop on nematode pheromones. Curr. Biol. 23(1): 83-86.
All images with exception of the second image are listed as free to reuse by Google. The second image is original artwork by the author.